How do you design and optimize chemical processes? This can be a difficult task, but it is necessary if you want to achieve the best possible results.
Process Design and Optimization is an essential discipline for anyone working in the chemical industry. It involves understanding the chemical reaction mechanism, designing the plant or refinery for optimal production, and optimizing processing conditions to achieve maximum efficiency.
There are a number of factors to consider when designing and optimizing chemical processes. In this guide, we will cover all those important aspects of design and optimization. By understanding these, you will be able to create efficient production arrangements that meet your specific needs.
Let’s take a look at the table of content ahead:
- Chemical Process Design and Optimization: An Overview
- (I) Importance of Chemical Process Design and Optimization
- (II) Chemical Process Design
- (III) Chemical Process Optimization
- (IV) Factors Affecting Chemical Process Design and Optimization
- (V) Future Trends in Chemical Process Design and Optimization
- (VI) Wrapping Up
- How Deskera Can Assist You?
Let's get started!
Chemical Process Design and Optimization: An Overview
Chemical process design and optimization is the process of creating and improving the manufacturing processes for chemicals and related products.
The ultimate goal of chemical process design is to develop a cost-effective and safe process that can produce high-quality products at a high yield. Moreover, optimization involves analyzing and improving an existing process to make it more efficient, cost-effective, and environmentally sustainable.
Chemical process design involves several stages, including conceptual design, process development, detailed design, construction, and operation. The first step in chemical process design is to define the goals and objectives of the project, including the desired product properties, production rate, and cost.
The process flow diagram is then developed, which outlines the sequence of operations required to produce the desired product. This includes the equipment needed, the raw materials required, and the process conditions.
Design tools and software are also used to aid in the chemical process design process. For example, simulation software can be used to predict the behavior of the process and to identify potential issues before the process is implemented. Other tools include process hazard analysis, risk assessment, and environmental impact assessment.
Chemical process optimization involves identifying and addressing the factors that affect the performance of the process, such as yield, energy consumption, and product quality.
Furthermore, various techniques can be used to optimize the process, including statistical methods, mathematical programming, and artificial intelligence and machine learning.
For example, statistical methods can be used to identify the variables that have the most significant impact on process performance.
Mathematical programming techniques can be used to optimize the process parameters, such as temperature and pressure, to achieve the desired output.
Artificial intelligence and machine learning algorithms can be used to develop models to predict the process behavior and optimize the process performance.
Factors that affect chemical process design and optimization include the physical and chemical properties of the raw materials, environmental and safety considerations, economic feasibility, and product quality and yield.
In recent years, there has been an increasing focus on integrating sustainability and circular economy principles into chemical process design and optimization. This involves designing processes that minimize waste and energy consumption and promote the reuse of materials.
All in all, chemical process design and optimization is a crucial aspect of the chemical manufacturing industry. It involves creating and improving processes to produce high-quality products efficiently and cost-effectively while also considering environmental and safety concerns. By continually improving these processes, chemical manufacturers can achieve greater sustainability, efficiency, and profitability.
(I) Importance of Chemical Process Design and Optimization
Chemical process design and optimization are essential for the chemical manufacturing industry. Here are some reasons why:
Cost reduction: Chemical process design and optimization can help reduce production costs by identifying and eliminating inefficiencies in the production process. By optimizing the process, manufacturers can produce more with fewer resources, reducing the cost of production.
Improved product quality: By optimizing the process, manufacturers can improve the quality of the product. The process can be designed to produce products that meet specific quality standards, resulting in fewer defects and customer complaints.
Increased safety: Chemical process design and optimization can help ensure the safety of workers and the surrounding environment. By identifying potential hazards and mitigating them during the design stage, manufacturers can reduce the risk of accidents during production.
Environmental sustainability: Chemical process design and optimization can help reduce the impact of production on the environment. By designing processes that minimize waste and energy consumption and promote the reuse of materials, manufacturers can reduce their carbon footprint and contribute to a more sustainable future.
Competitive advantage: Chemical process design and optimization can give manufacturers a competitive advantage by improving their efficiency, quality, and sustainability. This can help them gain market share and increase profitability.
Innovation: Chemical process design and optimization can drive innovation in the industry by promoting the use of new technologies and approaches. This can lead to the development of new products and processes that are more efficient and sustainable.
Ultimately, chemical process design and optimization play a crucial role in the chemical manufacturing industry. They can help reduce production costs, improve product quality, increase safety, promote environmental sustainability, provide a competitive advantage, and drive innovation. Manufacturers that prioritize chemical process design and optimization can achieve greater efficiency, profitability, and sustainability.
(II) Chemical Process Design
Chemical process design involves the creation of a manufacturing process that can produce a chemical or related product in a cost-effective and safe manner. The process design involves several stages, including conceptual design, process development, detailed design, construction, and operation.
Preliminary Design: In this stage, the preliminary design is developed based on the conceptual design and process development stages. The preliminary design includes equipment specifications, process control systems, safety features, and a preliminary piping and instrumentation diagram (P&ID).
Conceptual Design: In this stage, the goals and objectives of the project are defined. This includes the desired product properties, production rate, and cost. The process flow diagram is then developed, which outlines the sequence of operations required to produce the desired product. This includes the equipment needed, the raw materials required, and the process conditions.
Process Development: In this stage, the process is further developed and optimized. The process development stage involves laboratory experiments and testing to identify the most efficient and effective process. The process can be optimized by adjusting process conditions, such as temperature, pressure, and flow rates.
Detailed Design: In this stage, the process is designed in detail, including the equipment specifications, process control systems, and safety features. The detailed design stage also includes the development of a piping and instrumentation diagram (P&ID), which outlines the flow of materials and energy through the process.
Construction: In this stage, the process is constructed based on the detailed design. The construction stage involves the installation of equipment, piping, and instrumentation. Safety features are also installed to ensure the safe operation of the process.
Commissioning: In this stage, the process is tested and verified to ensure that it is ready for operation. The commissioning stage involves testing equipment, control systems, and safety systems. It also involves testing the process to ensure that it can produce the desired product at the desired quality and quantity.
Operation: In this stage, the process is operated to produce the desired product. The operation stage involves monitoring and controlling the process to ensure that it is running efficiently and safely. Regular maintenance and inspections are also conducted to ensure that the process is operating as designed.
Design tools and software are used to aid in the chemical process design process. For example, simulation software can be used to predict the behavior of the process and to identify potential issues before the process is implemented. Other tools include process hazard analysis, risk assessment, and environmental impact assessment.
Factors that affect chemical process design include the physical and chemical properties of the raw materials, environmental and safety considerations, economic feasibility, and product quality and yield. The design process must also consider the availability of resources, such as water and energy, and comply with local regulations and standards.
In conclusion, chemical process design is a critical aspect of the chemical manufacturing industry. It involves creating a cost-effective and safe process that can produce high-quality products efficiently. The design process includes several stages, including conceptual design, process development, detailed design, construction, and operation.
Design tools and software are used to aid in the design process, and several factors must be considered, including physical and chemical properties of raw materials, environmental and safety considerations, economic feasibility, and product quality and yield.
Process Flow Diagram (PFD)
A chemical process flow diagram (PFD) is a diagram that shows the various unit operations and processes involved in a chemical plant. The PFD provides a visual representation of the process flow and equipment used in the production of a chemical product.
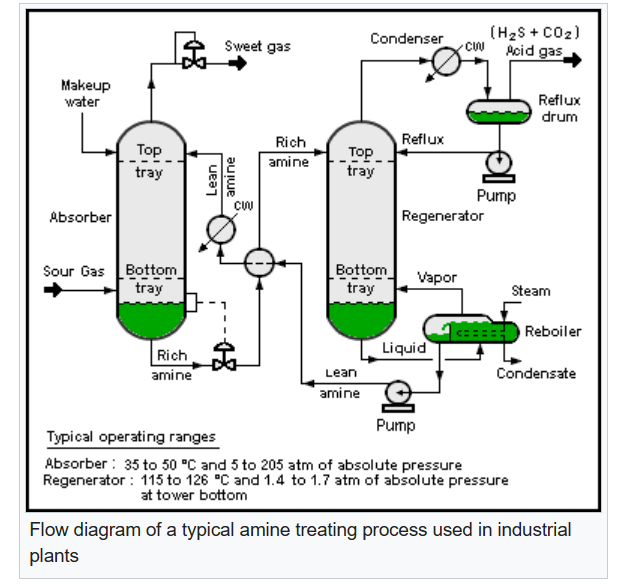
The PFD typically includes the following elements:
Major equipment: This includes the reactors, heat exchangers, pumps, and other major equipment used in the process.
Piping: The piping system that connects the various equipment and process units is shown in the PFD. The piping system may include valves, fittings, and other components.
Streams: The various streams of materials and energy are shown in the PFD. This includes the raw materials, intermediate products, and final products, as well as utility streams such as steam, water, and electricity.
Control systems: The control systems used to operate the process are shown in the PFD. This includes the instrumentation and control systems that monitor and control the process variables.
Safety systems: The safety systems used to protect the process and personnel are also shown in the PFD. This includes the relief valves, emergency shutdown systems, and other safety equipment.
The PFD is used to communicate the design of the process to various stakeholders, including process engineers, plant operators, and regulatory agencies. It provides a common language and visualization tool that can be used to identify potential problems and optimize the process design. The PFD is also used as a basis for developing the more detailed piping and instrumentation diagrams (P&ID) and the process control narratives.
The PFD is typically developed during the conceptual design phase of the process design, but it may be revised and updated throughout the design process as new information becomes available. As the design progresses from the conceptual to the detailed design phase, the PFD will become more detailed and will include additional information, such as equipment specifications and process control systems.
Design tools and software
There are several tools and software that are used in chemical process design to aid in the development, optimization, and analysis of a process. Here are some of the most commonly used tools and software:
Process Simulation Software: Process simulation software is used to create a virtual model of the chemical process. The software allows process engineers to predict the behavior of the process under different conditions, optimize the process design, and identify potential issues before the process is implemented. Examples of process simulation software include Aspen Plus, ChemCAD, and HYSYS.
Process Optimization Software: Process optimization software is used to optimize the design of a chemical process by identifying the optimal operating conditions and equipment design. The software can analyze large amounts of data and use algorithms to identify the optimal process parameters. Examples of process optimization software include GAMS, Lingo, and Excel Solver.
Process Safety Software: Process safety software is used to identify potential hazards and safety risks in a chemical process. The software can analyze process data and identify potential safety issues, such as the release of toxic gases or explosions. Examples of process safety software include PHA-Pro, Hazop+, and Process Safety Office.
Computational Fluid Dynamics (CFD) Software: CFD software is used to simulate the flow of fluids and heat transfer in a chemical process. The software can be used to optimize the design of heat exchangers, reactors, and other equipment used in the process. Examples of CFD software include ANSYS Fluent, COMSOL, and OpenFOAM.
Process Control Software: Process control software is used to control the operation of a chemical process by regulating process variables such as temperature, pressure, and flow rates. The software can optimize the control strategy and improve the efficiency and safety of the process. Examples of process control software include DeltaV, Honeywell Experion, and Siemens PCS7.
These tools and software can be used individually or in combination to aid in the chemical process design and optimization. They can help to reduce design time, improve process efficiency and safety, and minimize costs. However, it is important to note that these tools and software should be used in conjunction with the expertise of process engineers and other professionals to ensure that the design is safe, efficient, and cost-effective.
(III) Chemical Process Optimization
Chemical process optimization is the process of improving the efficiency and profitability of a chemical process by identifying and implementing changes that increase the yield of the desired product, reduce waste and energy consumption, and improve process safety. The goal of process optimization is to improve the process performance without compromising product quality or safety.
Process optimization involves the use of various techniques and tools to analyze and improve the process. These techniques can include:
Statistical Analysis: Statistical methods such as Design of Experiments (DOE) and statistical process control (SPC) can be used to identify factors that affect the process performance and optimize the process parameters.
Process Simulation: Process simulation tools can be used to create a virtual model of the process and analyze different scenarios to identify the optimal operating conditions.
Process Monitoring: Process monitoring tools such as sensors and control systems can be used to collect real-time data on the process performance and identify areas for improvement.
Process Control: Advanced process control (APC) systems can be used to optimize the control of the process variables and improve process efficiency.
Lean Six Sigma: Lean Six Sigma is a methodology that combines lean manufacturing and Six Sigma techniques to reduce waste and defects in the process and improve process efficiency.
The benefits of chemical process optimization include increased production capacity, improved product quality, reduced energy consumption and waste generation, and improved process safety. Process optimization can also lead to cost savings and increased profitability.
However, it is important to note that process optimization is an ongoing process and requires continuous monitoring and improvement. As the process conditions and requirements change over time, the process must be re-optimized to maintain optimal performance.
Techniques for chemical process optimization
There are several techniques that can be used for chemical process optimization. Here are some of the most commonly used techniques:
Statistical Process Control (SPC): SPC is a statistical technique that involves monitoring the process performance in real-time and taking corrective action when the process deviates from the desired performance. The technique involves collecting data on process variables such as temperature, pressure, and flow rates and using statistical methods to identify the sources of variation in the process.
Advanced Process Control (APC): APC is a set of techniques that use sophisticated algorithms and control strategies to optimize the process performance. APC involves the use of process models, sensors, and control systems to continuously monitor and adjust the process parameters in real-time to maintain optimal performance.
Lean Six Sigma: Lean Six Sigma is a methodology that combines lean manufacturing and Six Sigma techniques to reduce waste and defects in the process and improve process efficiency. The technique involves the identification and elimination of non-value-added activities and the use of statistical tools to reduce process variability.
Process Integration: Process integration involves the optimization of the entire process system, including the upstream and downstream processes. The technique involves the identification and elimination of inefficiencies in the process, such as excessive energy consumption or waste generation.
Dynamic Optimization: Dynamic optimization involves the optimization of the process performance under varying operating conditions. The technique involves the use of mathematical models and control strategies to optimize the process performance in real-time.
The selection of the appropriate technique for chemical process optimization depends on several factors, including the complexity of the process, the availability of data and resources, and the desired outcomes. The techniques can be used individually or in combination to achieve the desired results.
Statistical methods: Statistical methods are widely used in chemical process optimization to analyze process data and identify factors that affect the process performance. Here are some of the commonly used statistical methods for chemical process optimization:
Design of Experiments (DOE): DOE is a statistical technique that involves varying the process parameters systematically to identify the optimal settings for maximum yield and quality of the product. The technique involves selecting the most important process variables, defining their ranges, and creating a series of experiments to identify the best combination of process parameters. The results of the experiments are analyzed using statistical methods to identify the optimal process settings.
Response Surface Methodology (RSM): RSM is a statistical technique that is used to model the relationship between the process parameters and the response variables. The technique involves creating a mathematical model that describes the process performance as a function of the process parameters. The model is used to predict the process performance under different operating conditions and identify the optimal process settings.
Multivariate Analysis: Multivariate analysis is a statistical technique that is used to identify patterns and relationships in large datasets. The technique involves analyzing multiple variables simultaneously to identify the factors that affect the process performance. The results of the analysis can be used to develop a predictive model of the process performance and identify the optimal process settings.
Principal Component Analysis (PCA): PCA is a statistical technique that is used to reduce the dimensionality of the dataset by identifying the most important variables that explain the variation in the data. The technique involves transforming the original variables into a set of new variables that are uncorrelated and explain most of the variation in the data. The results of the analysis can be used to identify the key process variables and optimize the process performance.
The selection of the appropriate statistical method for chemical process optimization depends on several factors, including the complexity of the process, the availability of data, and the desired outcomes. The statistical methods can be used individually or in combination to achieve the desired results.
Mathematical programming: Mathematical programming is a technique used for chemical process optimization that involves the use of mathematical models to identify the optimal process settings.
Furthermore, the technique involves formulating a mathematical optimization problem that describes the process performance as a function of the process variables. The objective function of the problem is to maximize or minimize a specific performance metric, such as yield, throughput, or cost, while satisfying a set of constraints.
There are several types of mathematical programming techniques used for chemical process optimization, including:
Linear Programming (LP): LP is a mathematical programming technique that is used to optimize a linear objective function subject to a set of linear constraints. LP is widely used in chemical process optimization to optimize process performance and minimize costs.
Mixed Integer Linear Programming (MILP): MILP is an extension of LP that allows for integer variables in the optimization problem. The technique is used when the process variables can only take on integer values, such as the number of production runs or the number of machines in a manufacturing process.
Nonlinear Programming (NLP): NLP is a mathematical programming technique used to optimize a nonlinear objective function subject to nonlinear constraints. NLP is commonly used in chemical process optimization to model complex nonlinear systems, such as chemical reactions and fluid dynamics.
Mixed Integer Nonlinear Programming (MINLP): MINLP is an extension of NLP that allows for integer variables in the optimization problem. The technique is used when the process variables can only take on integer values and the objective function or constraints are nonlinear.
Dynamic Programming (DP): DP is a mathematical programming technique used to optimize a process over time. The technique is used when the process performance is affected by time-varying parameters, such as demand or raw material availability.
The selection of the appropriate mathematical programming technique for chemical process optimization depends on several factors, including the complexity of the process, the availability of data, and the desired outcomes. The mathematical programming techniques can be used individually or in combination with other optimization techniques to achieve the desired results.
Artificial Intelligence and Machine Learning
Artificial intelligence (AI) and machine learning (ML) techniques are increasingly being used in chemical process design and optimization to improve process efficiency, reduce costs, and increase product quality. These techniques involve the use of advanced algorithms and models to analyze large datasets and identify patterns and relationships in the data.
Here are some of the commonly used AI and ML techniques for chemical process design and optimization:
Artificial Neural Networks (ANNs): ANNs are computational models inspired by the structure and function of the human brain. ANNs can be trained on large datasets to identify complex patterns and relationships in the data. In chemical process design and optimization, ANNs can be used to model the relationship between process variables and the process performance, and to identify the optimal process settings for maximum yield, quality, and efficiency.
Support Vector Machines (SVMs): SVMs are a type of supervised ML algorithm used for classification and regression analysis. In chemical process design and optimization, SVMs can be used to predict the process performance under different operating conditions, and to identify the optimal process settings for maximum yield and quality.
Genetic Algorithms (GAs): GAs are a type of optimization algorithm inspired by the process of natural selection. In chemical process design and optimization, GAs can be used to identify the optimal process settings by simulating the process of natural selection and evolution.
Particle Swarm Optimization (PSO): PSO is an optimization technique inspired by the behavior of swarms and flocks in nature. In chemical process design and optimization, PSO can be used to identify the optimal process settings by simulating the behavior of a swarm of particles searching for the global optimum.
Deep Learning (DL): DL is a type of ML algorithm that uses deep neural networks with multiple layers to analyze complex datasets. In chemical process design and optimization, DL can be used to model the relationship between the process variables and the process performance, and to identify the optimal process settings for maximum yield and quality.
The selection of the appropriate AI and ML technique for chemical process design and optimization depends on several factors, including the complexity of the process, the availability of data, and the desired outcomes. The AI and ML techniques can be used individually or in combination with other optimization techniques to achieve the desired results.
Case studies on chemical process optimization
Here are a few case studies that illustrate the use of different optimization techniques for chemical process optimization:
Optimization of a Chemical Reactor Process Using Nonlinear Programming (NLP):
A case study published in the Journal of Process Control details the optimization of a chemical reactor process using NLP. The process involved the production of an intermediate chemical using a continuous stirred-tank reactor.
The objective was to maximize the yield of the intermediate chemical while minimizing the production cost. The optimization problem was formulated using a nonlinear objective function and constraints, and the optimization was performed using an NLP solver. The optimized process resulted in a significant improvement in yield and cost savings.
Optimization of a Distillation Column Using Genetic Algorithms (GAs):
A case study published in Chemical Engineering and Processing: Process Intensification describes the optimization of a distillation column using GAs. The distillation column was used to separate a mixture of organic compounds, and the objective was to maximize the purity of the product while minimizing the energy consumption.
The optimization problem was formulated using a genetic algorithm, and the optimal process settings were identified by simulating the process of natural selection and evolution. The optimized process resulted in a significant improvement in purity and energy savings.
Optimization of a Chemical Process Using Artificial Neural Networks (ANNs):
A case study published in the International Journal of Industrial Chemistry describes the optimization of a chemical process using ANNs. The process involved the production of a pharmaceutical intermediate using a batch reactor.
The objective was to maximize the yield of the intermediate while minimizing the production cost. Moreover, the optimization problem was formulated using an ANN model, and the optimal process settings were identified by training the ANN on historical data and performing a sensitivity analysis. The optimized process resulted in a significant improvement in yield and cost savings.
Optimization of a Crystallization Process Using Particle Swarm Optimization (PSO):
A case study published in the Journal of Chemical Technology and Biotechnology describes the optimization of a crystallization process using PSO.
The process involved the production of a high-value intermediate using a batch crystallizer. The objective was to maximize the yield of the intermediate while maintaining the desired crystal size distribution.
The optimization problem was formulated using a PSO algorithm, and the optimal process settings were identified by simulating the behavior of a swarm of particles searching for the global optimum. The optimized process resulted in a significant improvement in yield and crystal size distribution.
Ultimately, these case studies demonstrate the effectiveness of different optimization techniques for chemical process optimization and highlight the importance of selecting the appropriate technique for the specific process and desired outcomes.
(IV) Factors Affecting Chemical Process Design and Optimization
There are several factors that can affect chemical process design and optimization, including:
Process Constraints: These are limitations or requirements imposed by the process itself, such as feedstock availability, reaction kinetics, thermodynamics, and product specifications.
Furthermore, the process constraints can significantly impact the design and optimization of the chemical process, as they need to be carefully considered and accommodated in the process design and optimization.
Equipment Selection and Sizing: The choice of equipment and its sizing can significantly impact the design and optimization of the chemical process. The equipment selection and sizing depend on several factors, such as the feedstock properties, reaction kinetics, operating conditions, and desired product specifications. A careful evaluation of the equipment options and sizing can help optimize the process design and minimize the production cost.
Energy Efficiency: Energy efficiency is a critical factor in chemical process design and optimization, as it can significantly impact the production cost and environmental impact of the process.
Moreover, the selection of energy-efficient equipment, process integration, and optimization of energy consumption are essential for minimizing the production cost and reducing the environmental footprint of the process.
Safety and Environmental Regulations: Safety and environmental regulations can significantly impact the design and optimization of chemical processes. Compliance with safety and environmental regulations can affect the process design, equipment selection, operating conditions, and waste treatment options. A careful consideration of safety and environmental regulations is essential for ensuring the safe and sustainable operation of the process.
Cost and Profitability: The production cost and profitability are critical factors in chemical process design and optimization. The cost of feedstocks, energy, labor, and equipment can significantly impact the production cost and profitability of the process. An optimization of the process design and operating conditions can help minimize the production cost and maximize the profitability of the process.
Process Flexibility: The process flexibility refers to the ability of the process to adapt to changes in feedstock availability, product demand, and market conditions. A flexible process design can help minimize the production cost and maximize the profitability of the process by allowing the process to adjust to changes in the operating conditions and market conditions.
These factors highlight the importance of a comprehensive and systematic approach to chemical process design and optimization, considering the interplay between different factors and the desired process outcomes.
Physical and Chemical Properties of Raw Materials
The physical and chemical properties of raw materials play a critical role in chemical processes. These properties can affect the process design and optimization, as well as the product quality and safety. Some of the important physical and chemical properties of raw materials in chemical processes include:
Physical State: The physical state of the raw material (solid, liquid, or gas) can significantly impact the process design and equipment selection. For example, liquid or gas feedstocks may require different pumping and piping systems compared to solid feedstocks. The physical state can also affect the reaction kinetics and thermodynamics, which can impact the process optimization.
Density: The density of the raw material can affect the storage and transportation requirements, as well as the equipment sizing. High-density feedstocks may require larger storage tanks and piping systems, while low-density feedstocks may require additional handling and processing to achieve the desired product concentration.
Viscosity: The viscosity of the raw material can impact the flow characteristics and pumping requirements. High viscosity feedstocks may require additional heating or mixing to reduce the viscosity and improve the process efficiency.
Chemical Composition: The chemical composition of the raw material can affect the reaction kinetics and thermodynamics, as well as the product quality and safety. The presence of impurities or contaminants can affect the process efficiency and product purity and may require additional processing or treatment to achieve the desired product specifications.
Reactivity: The reactivity of the raw material can impact the process safety and equipment selection. Highly reactive feedstocks may require additional safety measures, such as special storage or handling requirements, and may also require specialized equipment to ensure safe and efficient processing.
Solubility: The solubility of the raw material can impact the process efficiency and product quality. Feedstocks that are not soluble in the reaction medium may require additional processing or treatment to achieve the desired product concentration.
Heat Capacity: The heat capacity of the raw material can impact the energy requirements and process efficiency. High heat capacity feedstocks may require additional heating or cooling to maintain the desired reaction temperature and optimize the process efficiency.
Therefore, understanding the physical and chemical properties of raw materials is crucial for the design and optimization of chemical processes. A comprehensive evaluation of these properties can help optimize the process design, reduce the production cost, and improve the product quality and safety.
Environmental and Safety Considerations
Chemical processes have the potential to cause environmental harm and pose safety risks to workers and the public. Therefore, it is essential to consider environmental and safety factors during the design and optimization of chemical processes. Here are some key considerations:
Hazardous Materials: Many chemical processes involve hazardous materials, such as toxic chemicals, flammable liquids, and explosive compounds. It is important to identify and assess the potential risks associated with these materials, and to implement appropriate safety measures to prevent accidents and protect workers and the environment.
Pollution Prevention: Chemical processes can generate waste streams that can be harmful to the environment if not properly managed. It is essential to design the process to minimize waste generation and to implement appropriate waste treatment and disposal methods to ensure compliance with environmental regulations.
Energy Efficiency: Chemical processes can consume significant amounts of energy, which can contribute to greenhouse gas emissions and climate change. It is important to consider energy efficiency during the process design and optimization, and to identify opportunities for energy conservation and renewable energy use.
Process Safety: Chemical processes can pose significant safety risks to workers and the public. It is important to assess the potential hazards associated with the process and to implement appropriate safety measures, such as process controls, personal protective equipment, and emergency response procedures.
Process Monitoring: Chemical processes should be monitored to ensure that they are operating within safe and efficient parameters. Monitoring can include process control instrumentation, process performance measurements, and environmental monitoring.
Regulatory Compliance: Chemical processes are subject to numerous environmental, health, and safety regulations. It is important to ensure that the process design and operation comply with these regulations and to maintain appropriate documentation and reporting to demonstrate compliance.
In conclusion, environmental and safety considerations are critical for the design and optimization of chemical processes. By addressing these factors, it is possible to reduce the environmental impact of the process, ensure worker and public safety, and comply with regulatory requirements.
Economic Feasibility
In addition to considering the physical, chemical, environmental, and safety factors of a chemical process, economic feasibility is also an important consideration. Here are some key factors to consider:
Capital Costs: The capital cost of a chemical process includes the cost of equipment, infrastructure, and construction. The feasibility of a project will depend on whether the expected revenue generated by the process is sufficient to cover the capital costs.
Operating Costs: The operating cost of a chemical process includes the cost of raw materials, utilities, labor, and maintenance. The feasibility of a project will depend on whether the expected revenue generated by the process is sufficient to cover the operating costs.
Revenue: The revenue generated by a chemical process will depend on the market demand for the product, the price at which the product can be sold, and the quantity of product that can be produced.
Return on Investment: The return on investment (ROI) is a key metric for determining the economic feasibility of a chemical process. ROI is calculated as the ratio of the net income generated by the process to the capital invested in the process. A high ROI is typically required for a project to be considered economically feasible.
Risk Analysis: Economic feasibility also involves assessing the risks associated with the project, such as market demand volatility, raw material price fluctuations, and regulatory changes. Risk analysis helps to identify potential threats to the financial viability of the project and to develop strategies to mitigate these risks.
Life Cycle Cost Analysis: Life cycle cost analysis involves assessing the total cost of the chemical process over its entire life cycle, including capital costs, operating costs, maintenance costs, and disposal costs. This analysis helps to identify opportunities for cost savings and to make informed decisions about the design and operation of the process.
Ultimately, economic feasibility is an important consideration in the design and optimization of chemical processes. By assessing capital costs, operating costs, revenue, ROI, risk, and life cycle costs, it is possible to determine whether a project is financially viable and to make informed decisions about the design and operation of the process.
Product Quality and Yield
Product quality and yield are critical factors in chemical processes as they directly impact the profitability of the process. Here are some key considerations for ensuring high product quality and yield:
Raw Material Quality: The quality of the raw materials used in the process can greatly impact the quality and yield of the final product. It is important to source high-quality raw materials and to test them prior to use to ensure that they meet the necessary specifications.
Process Conditions: The process conditions, such as temperature, pressure, and residence time, can greatly impact the quality and yield of the final product. It is important to optimize these conditions to ensure that the desired product quality and yield are achieved.
Catalysts and Additives: The use of catalysts and additives can help to improve product quality and yield. For example, a catalyst can help to increase the reaction rate and selectivity, while an additive can help to improve product purity or prevent unwanted side reactions.
Process Monitoring and Control: Process monitoring, and control can help to ensure that the process is operating within the desired parameters and that the desired product quality and yield are being achieved. This can involve using sensors to measure key process variables, such as temperature and pressure, and adjusting process conditions as necessary.
Separation and Purification: Separation and purification steps are often necessary to achieve the desired product quality and yield. These steps can include distillation, chromatography, and filtration, among others.
Waste Management: Proper waste management is also critical for ensuring high product quality and yield. Waste streams must be properly treated and disposed of to prevent contamination of the final product and to comply with environmental regulations.
Therefore, product quality and yield are critical factors in chemical processes that needs to be considered. By ensuring high raw material quality, optimizing process conditions, using catalysts and additives, monitoring and controlling the process, implementing separation and purification steps, and properly managing waste, it is possible to achieve the desired product quality and yield and to maximize the profitability of the process.
(V) Future Trends in Chemical Process Design and Optimization
The field of chemical process design and optimization is constantly evolving, driven by advancements in technology and changing economic, environmental, and regulatory pressures.
Emerging Technologies and Trends
There are several emerging technologies and trends in chemical process design and optimization that have the potential to revolutionize the industry. Here are a few examples:
Process Intensification: Process intensification involves developing more efficient, compact, and integrated processes that require less energy and resources. This can be achieved through techniques such as microreactors, membrane reactors, and intensified heat transfer.
Green Chemistry: Green chemistry involves developing chemical processes that are more sustainable and environmentally friendly. This can involve using renewable feedstocks, reducing waste, and minimizing the use of hazardous chemicals.
Artificial Intelligence and Machine Learning: Artificial intelligence and machine learning can be used to optimize chemical processes by analyzing large datasets and identifying patterns that would be difficult to detect with traditional methods.
Advanced Materials: Advanced materials, such as nanomaterials and biomaterials, have the potential to improve the performance of chemical processes. For example, nanomaterials can be used as catalysts to increase reaction rates and selectivity.
3D Printing: 3D printing can be used to create custom parts and equipment for chemical processes, allowing for greater flexibility and customization.
Modular and Flexible Manufacturing: Modular and flexible manufacturing involves developing processes that can be easily modified or scaled up or down to meet changing demand. This can be achieved through techniques such as modular process design and flexible manufacturing platforms.
Continuous Manufacturing: Continuous manufacturing involves developing processes that operate continuously, as opposed to traditional batch processes. This can result in higher efficiency and productivity, as well as improved product quality.
Overall, there are several emerging technologies and trends in chemical process design and optimization that have the potential to improve efficiency, sustainability, and performance. By embracing these new technologies and trends, chemical manufacturers can stay competitive and meet the evolving needs of the industry and society.
Integration of Sustainability and Circular Economy Principles
Integration of sustainability and circular economy principles in chemical process design and optimization has become increasingly important in recent years.
The goal of sustainability is to minimize the negative impact of chemical processes on the environment, society, and economy, while circular economy principles focus on reducing waste and creating a closed-loop system that maximizes resource efficiency.
Here are some ways in which sustainability and circular economy principles can be integrated into chemical process design and optimization:
Life Cycle Assessment: Life cycle assessment (LCA) is a tool that can be used to evaluate the environmental impact of chemical processes throughout their entire life cycle, from raw material extraction to end-of-life disposal. By using LCA, designers can identify opportunities to reduce the environmental impact of chemical processes, such as by using renewable energy sources or reducing waste generation.
Green Chemistry: Green chemistry involves developing chemical processes that are more sustainable and environmentally friendly. This can involve using renewable feedstocks, reducing waste, and minimizing the use of hazardous chemicals.
Process Intensification: Process intensification can help to reduce the environmental impact of chemical processes by minimizing the use of energy and resources. By developing more efficient, compact, and integrated processes, designers can reduce the number of raw materials and energy required, resulting in lower emissions and waste generation.
Closed-Loop Systems: Closed-loop systems, which involve reusing or recycling materials and by-products, can help to reduce waste and increase resource efficiency. For example, by developing processes that use waste streams as feedstocks or by recycling materials, designers can reduce the amount of waste that is sent to landfills and minimize the need for virgin materials.
Renewable Energy Sources: By using renewable energy sources, such as wind or solar power, designers can reduce the environmental impact of chemical processes and decrease their reliance on non-renewable resources.
Circular Design: Circular design involves developing processes that are designed to maximize resource efficiency and minimize waste generation. By designing products and processes with end-of-life considerations in mind, designers can create closed-loop systems that minimize waste and increase resource efficiency.
By integrating sustainability and circular economy principles into chemical process design and optimization, designers can create processes that are more efficient, sustainable, and environmentally friendly. This can help to reduce the negative impact of chemical processes on the environment and society, while also improving the bottom line for chemical manufacturers.
Role of Digitalization and Industry 4.0
Digitalization and Industry 4.0 are transforming the chemical process industry by enabling increased automation, optimization, and efficiency. Here are some ways in which digitalization and Industry 4.0 are impacting chemical process design and optimization:
Process Control and Automation: Digitalization and Industry 4.0 technologies are enabling increased process control and automation, which can help to optimize chemical processes and reduce variability. This can involve the use of sensors and data analytics to monitor and control process variables in real-time, allowing for faster and more precise adjustments to process conditions.
Predictive Maintenance: Predictive maintenance involves using data analytics and machine learning to predict when maintenance will be required, allowing for more efficient and cost-effective maintenance scheduling. Moreover, by using sensors and data analytics to monitor equipment performance, chemical manufacturers can identify potential issues before they occur, reducing downtime and maintenance costs.
Simulation and Modeling: Digitalization and Industry 4.0 technologies are enabling more advanced simulation and modeling of chemical processes, allowing for more accurate and efficient process design and optimization. This can involve the use of computational fluid dynamics (CFD) and other simulation tools to model process conditions and optimize process parameters.
Big Data Analytics: Big data analytics involves using advanced data analytics techniques to identify patterns and insights in large datasets. By analyzing data from sensors, equipment, and other sources, chemical manufacturers can identify opportunities to optimize processes and improve efficiency.
Digital Twins: Digital twins in chemical manufacturing involve creating a virtual replica of a physical process or system, allowing for real-time monitoring and optimization. By using sensors and data analytics to create a digital twin of a chemical process, manufacturers can identify opportunities to optimize process conditions and improve efficiency.
Collaborative Platforms: Digitalization and Industry 4.0 technologies are enabling more collaborative and connected work environments, allowing for increased communication and collaboration across different teams and departments. This can help to improve process design and optimization by enabling more efficient sharing of data and knowledge.
Overall, digitalization and Industry 4.0 are enabling chemical manufacturers to optimize processes, reduce costs, and improve efficiency. By leveraging advanced technologies such as process control and automation, predictive maintenance, simulation and modeling, big data analytics, digital twins, and collaborative platforms, chemical manufacturers can create more efficient, sustainable, and profitable processes.
Some Important Questions Associated with Chemical Process Design and Optimization
Following, we’ve discussed some crucial questions associated with chemical process design and optimization.
Que 1: Importance of continuous improvement in chemical process design and optimization
Ans: Continuous improvement is crucial in chemical process design and optimization for several reasons. First, chemical processes can be complex and involve many variables, making it difficult to achieve optimal results in a single attempt. By continually reviewing and improving the process, engineers can identify areas for improvement and optimize the process over time.
Furthermore, continuous improvement also enables chemical manufacturers to adapt to changes in the market, customer demand, and technology. By continuously improving processes, manufacturers can stay competitive and improve efficiency, productivity, and profitability.
Additionally, continuous improvement can help manufacturers identify and address safety and environmental concerns. As new information becomes available, manufacturers can update their processes to reduce risks and minimize their environmental impact.
Finally, continuous improvement is essential for meeting sustainability goals. As companies strive to reduce their carbon footprint and minimize waste, they must continually evaluate their processes and identify opportunities for improvement.
Overall, continuous improvement is critical for ensuring that chemical processes are efficient, safe, sustainable, and profitable. By continually reviewing and optimizing processes, manufacturers can stay competitive, meet regulatory requirements, and create more value for customers and stakeholders.
Que 2: Future directions and opportunities for research and development in this field
Ans: The field of chemical process design and optimization is continuously evolving, driven by emerging technologies, changing market demands, and increasing environmental and sustainability concerns. Here are some of the future directions and opportunities for research and development in chemical process design and optimization:
Integration of renewable energy sources: As renewable energy sources become more prevalent, there is an opportunity to integrate them into chemical process design and optimization. Researchers can explore ways to use renewable energy sources to power chemical processes, reduce carbon emissions, and improve process efficiency.
Advanced process control: Advanced process control techniques, such as model predictive control, can be used to optimize chemical processes in real-time, increasing efficiency, reducing waste, and improving product quality.
Process intensification: Process intensification involves developing more compact, efficient, and integrated chemical processes. This approach can lead to significant energy savings, reduced costs, and improved sustainability.
Sustainable chemistry: Sustainable chemistry involves designing chemical processes that minimize waste, reduce energy consumption, and use safer chemicals. This approach can help address environmental and sustainability concerns while maintaining or improving product quality.
Big data and analytics: Big data and analytics can be used to analyze vast amounts of process data and identify opportunities for optimization, improvement, and innovation.
Additive manufacturing: Additive manufacturing, or 3D printing, can be used to create complex parts and components for chemical processes, improving efficiency and reducing costs.
Multi-objective optimization: multi-objective optimization involves optimizing processes to achieve multiple objectives simultaneously, such as reducing costs, minimizing waste, and improving product quality.
Overall, the future of chemical process design and optimization is likely to focus on achieving greater sustainability, efficiency, and profitability through the integration of advanced technologies, renewable energy sources, and innovative process design and control strategies.
VI. Wrapping Up
In conclusion, chemical process design and optimization are critical for ensuring efficient and sustainable production of chemicals, materials, and products.
The design of a chemical process involves a series of stages, including conceptualization, process flow diagram development, equipment sizing, and process simulation, which must be carefully planned and executed to ensure a successful outcome.
Optimization techniques, including statistical methods, mathematical programming, and artificial intelligence, can be used to improve process efficiency, reduce costs, and minimize environmental impacts.
Several factors, including raw material properties, environmental and safety considerations, economic feasibility, and product quality and yield, must be considered during the chemical process design and optimization process. Emerging technologies, such as digitalization and Industry 4.0, are also transforming the chemical process industry by enabling increased automation, optimization, and efficiency.
It is essential for chemical engineers and process designers to stay up-to-date with emerging trends and technologies in chemical process design and optimization to remain competitive in the industry. By integrating sustainability and circular economy principles into chemical process design and optimization and leveraging advanced technologies, chemical manufacturers can create more efficient, sustainable, and profitable processes.
How Deskera Can Assist You?
Deskera's integrated financial planning tools allow investors to better plan their investments and track their progress. It can help investors make decisions faster and more accurately.
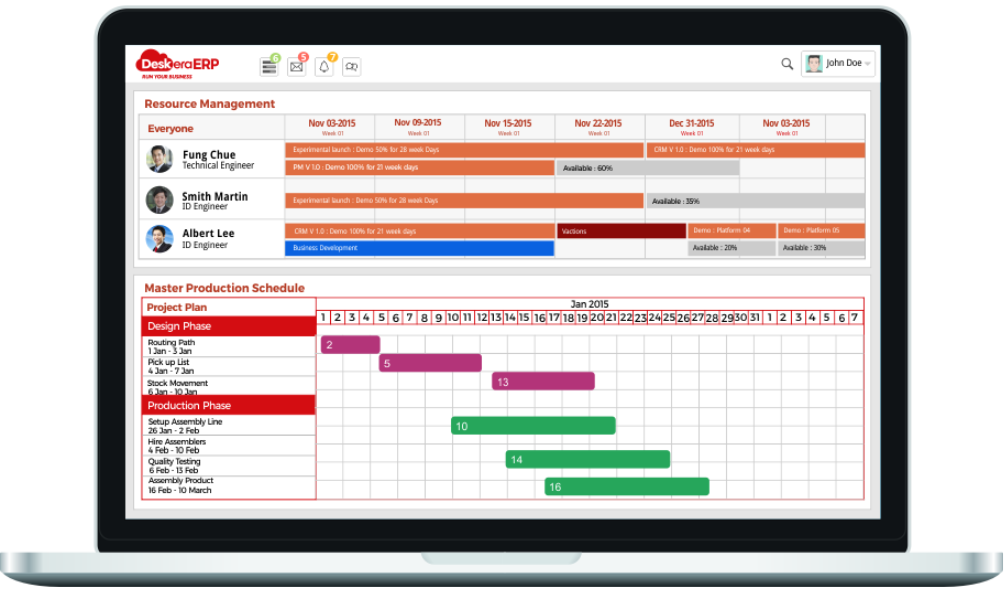
Deskera Books enables you to manage your accounts and finances more effectively. Maintain sound accounting practices by automating accounting operations such as billing, invoicing, and payment processing.
Deskera CRM is a strong solution that manages your sales and assists you in closing agreements quickly. It not only allows you to do critical duties such as lead generation via email, but it also provides you with a comprehensive view of your sales funnel.
Deskera People is a simple tool for taking control of your human resource management functions. The technology not only speeds up payroll processing but also allows you to manage all other activities such as overtime, benefits, bonuses, training programs, and much more. This is your chance to grow your business, increase earnings, and improve the efficiency of the entire production process.
Final Takeaways
We've arrived at the last section of this guide. Let's have a look at some of the most important points to remember:
- Chemical process design and optimization is the process of creating and improving the manufacturing processes for chemicals and related products. The ultimate goal of chemical process design is to develop a cost-effective and safe process that can produce high-quality products at a high yield.
- Cost reduction: Chemical process design and optimization can help reduce production costs by identifying and eliminating inefficiencies in the production process. By optimizing the process, manufacturers can produce more with fewer resources, reducing the cost of production.
- Process Development: In this stage, the process is further developed and optimized. The process development stage involves laboratory experiments and testing to identify the most efficient and effective process. The process can be optimized by adjusting process conditions, such as temperature, pressure, and flow rates.
- A chemical process flow diagram (PFD) is a diagram that shows the various unit operations and processes involved in a chemical plant. The PFD provides a visual representation of the process flow and equipment used in the production of a chemical product.
- Chemical process optimization is the process of improving the efficiency and profitability of a chemical process by identifying and implementing changes that increase the yield of the desired product, reduce waste and energy consumption, and improve process safety. The goal of process optimization is to improve the process performance without compromising product quality or safety.
- Mathematical programming is a technique used for chemical process optimization that involves the use of mathematical models to identify the optimal process settings. The technique involves formulating a mathematical optimization problem that describes the process performance as a function of the process variables.
- Many chemical processes involve hazardous materials, such as toxic chemicals, flammable liquids, and explosive compounds. It is important to identify and assess the potential risks associated with these materials, and to implement appropriate safety measures to prevent accidents and protect workers and the environment.
- Sustainable chemistry involves designing chemical processes that minimize waste, reduce energy consumption, and use safer chemicals. This approach can help address environmental and sustainability concerns while maintaining or improving product quality.
Related Articles
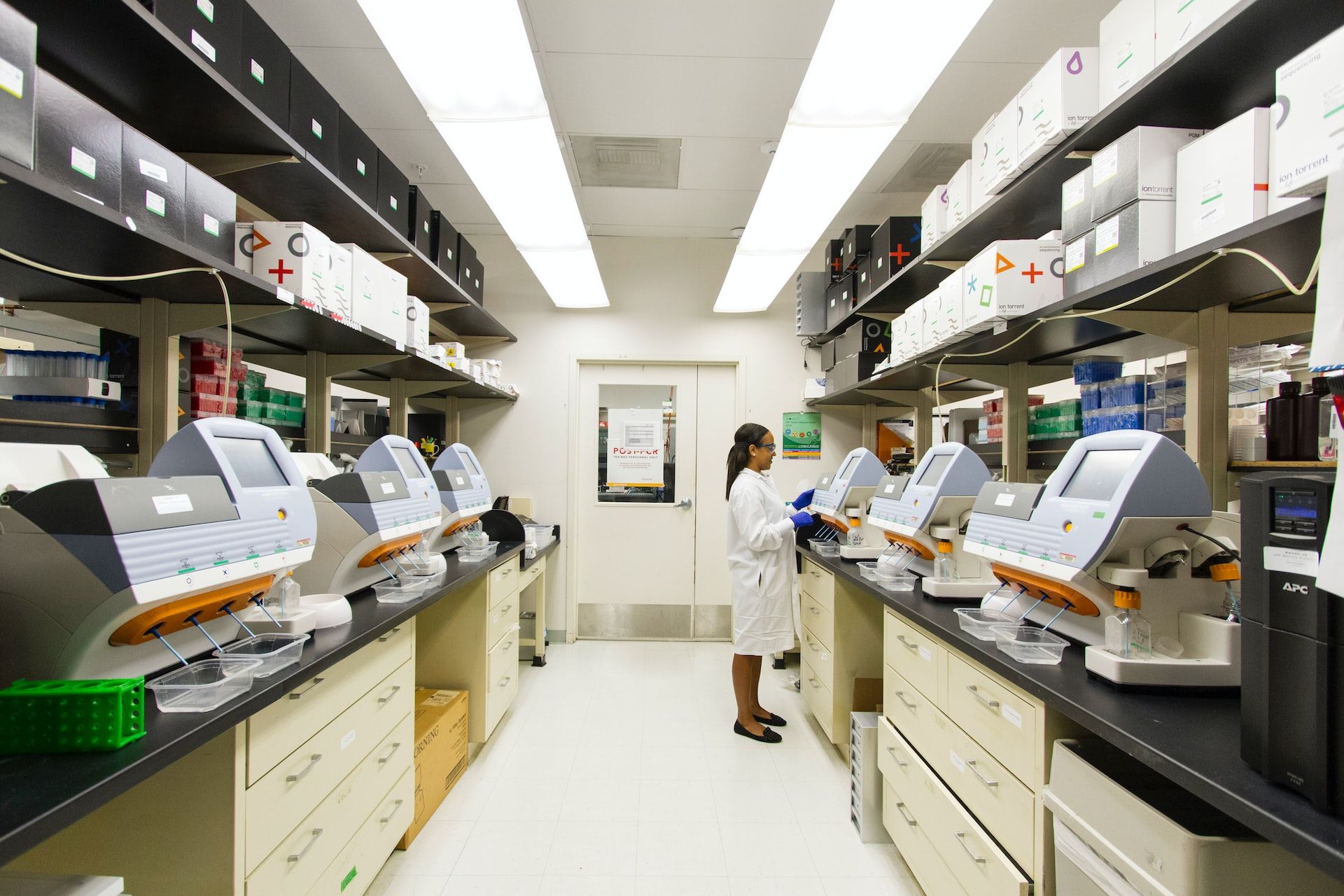
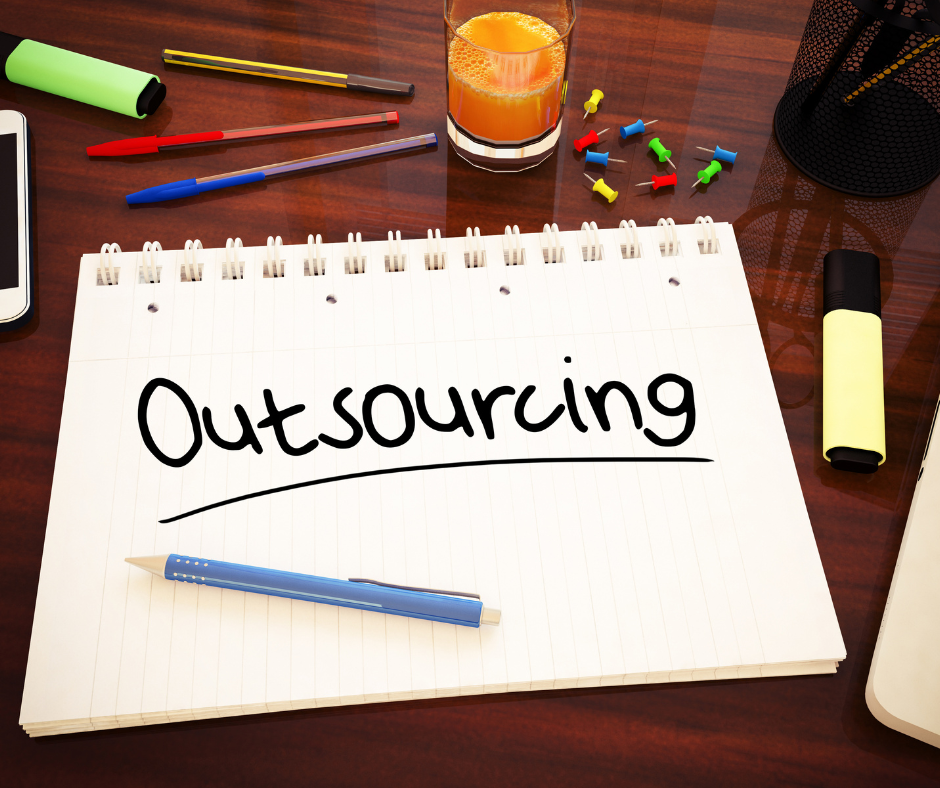
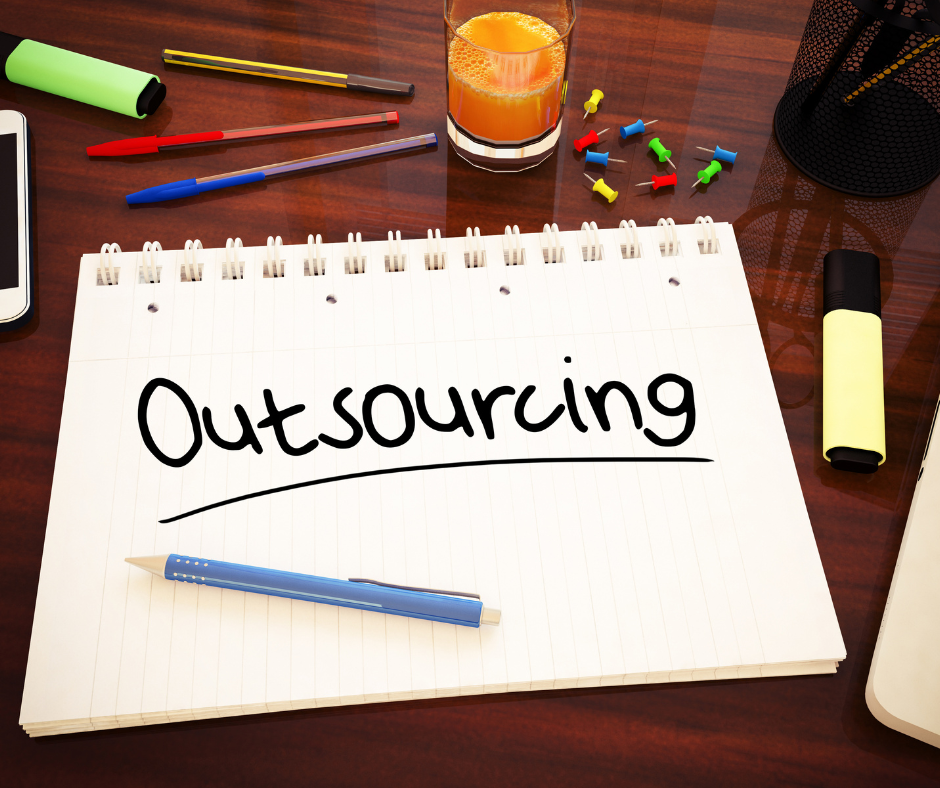
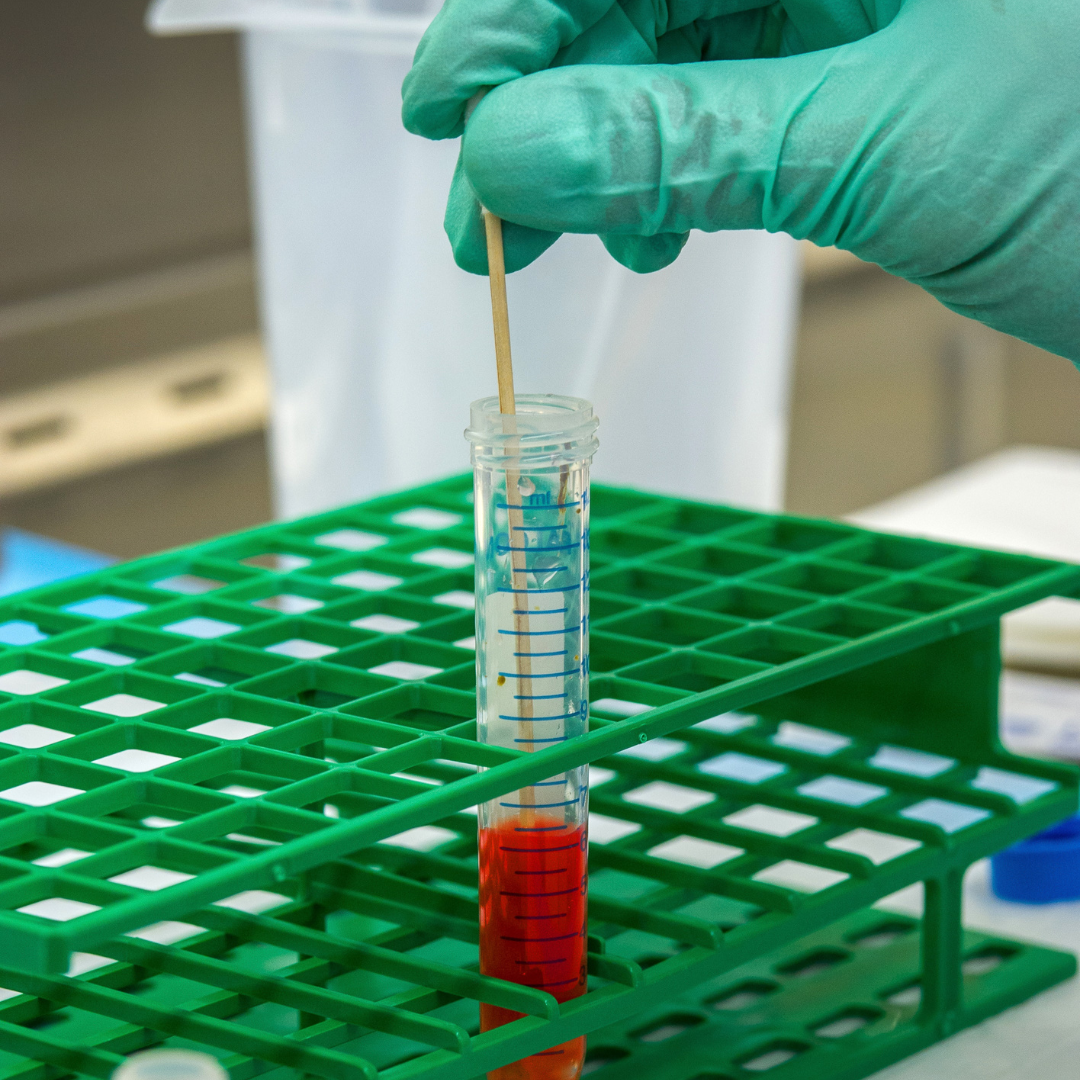
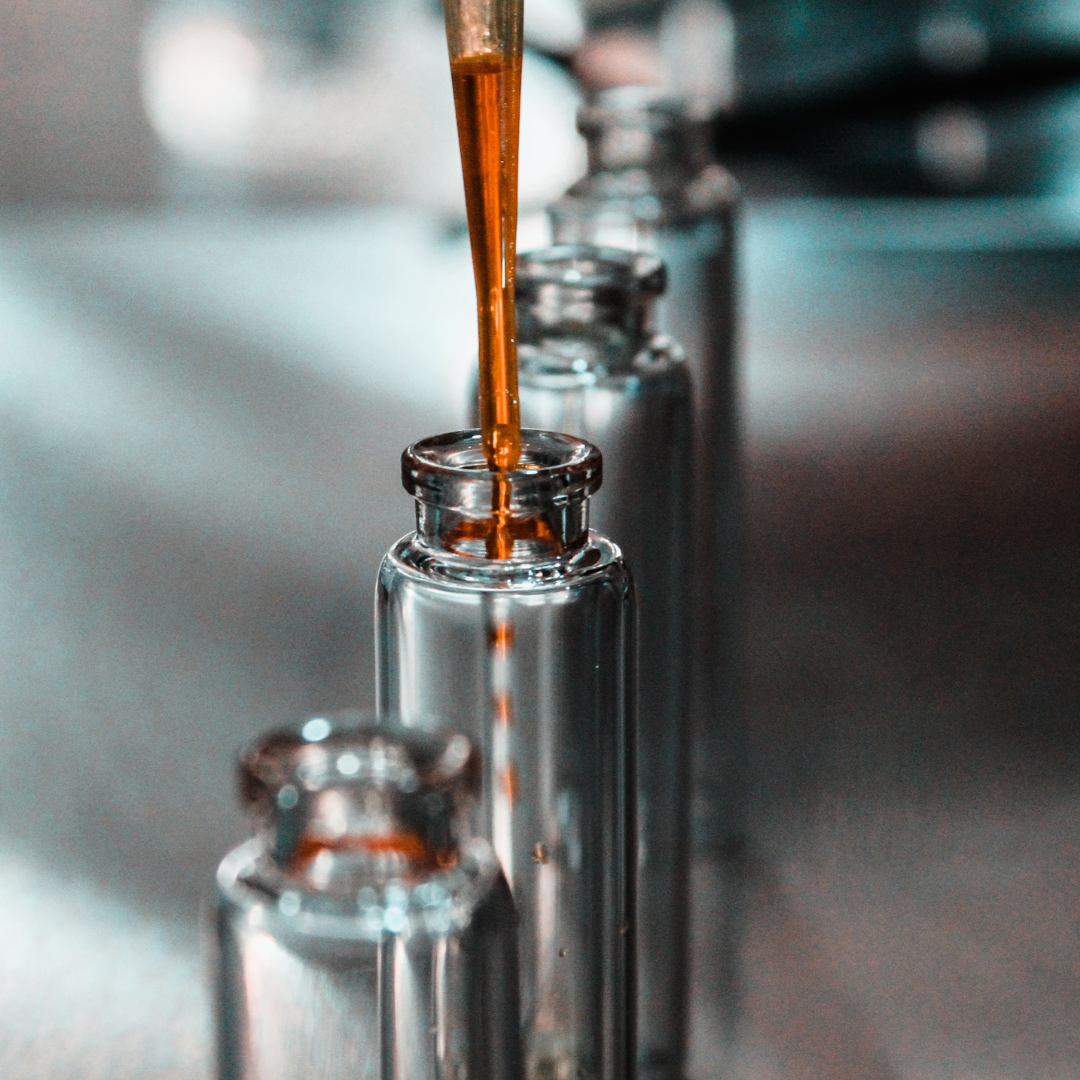